Pyrolysis is a pivotal thermal process in bio-oil production from biomass, involving the thermal decomposition of lignocellulosic materials at temperatures ranging from 400 to 600 °C in the absence of oxygen. This process primarily generates bio-oil, gas, and char, with fast pyrolysis maximizing bio-oil yield, potentially producing up to 75% bio-oil from feedstocks. Factors such as heating rates, particle size, and reactor design greatly impact the efficiency and quality of the bio-oil, which consists of 15-30 wt% water and a complex mixture of organic compounds. Understanding these variables is essential for optimizing bio-oil properties and applications. There's much more to explore on this technology's implications.
Key Takeaways
- Pyrolysis is the thermal decomposition of biomass at 400-600 °C in the absence of oxygen, producing bio-oil, gas, and char.
- Fast pyrolysis maximizes bio-oil yield by using high heating rates and short residence times, achieving up to 75% bio-oil.
- Bio-oil, a complex mixture with high oxygen content, requires upgrading for conventional fuel applications due to stability and energy density issues.
- Common biomass feedstocks for pyrolysis include agricultural residues and forestry waste, with low moisture content preferred for optimal yield.
- Pyrolysis reduces greenhouse gas emissions by 60-90% compared to fossil fuels, supporting renewable energy and waste valorization efforts.
Understanding Pyrolysis
What exactly is pyrolysis, and why should we care about it? Pyrolysis is the thermal decomposition of lignocellulosic biomass, occurring at elevated temperatures—typically between 400 and 600 °C—in the absence of oxygen. This process generates bio-oil, gas, and char, with fast pyrolysis being particularly significant for its high heating rates and short residence times, yielding up to 75% bio-oil. The conversion efficiency and quality of bio-oil are influenced by key operating parameters such as temperature, heating rates, and particle size. Bio-oil, which comprises 15-30 wt% water and various oxygenated organic compounds, often requires catalytic upgrading to enhance its properties, addressing issues like high acidity and viscosity, making it more suitable for conventional fuel applications.
Biomass Feedstocks for Pyrolysis
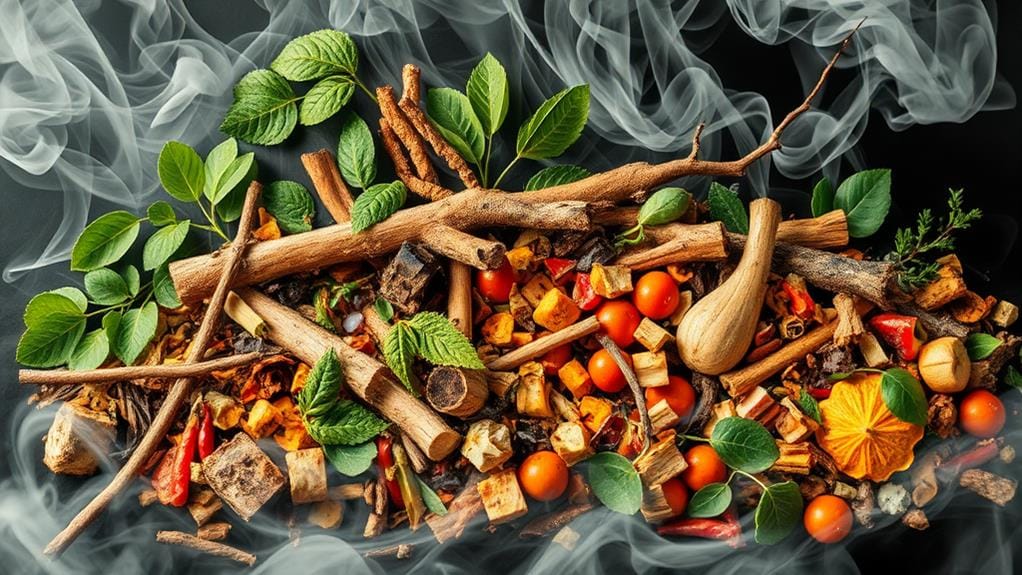
The diversity of biomass feedstocks available for pyrolysis offers exciting opportunities for bio-oil production. We can utilize agricultural residues, such as straw and corn stover, alongside forestry waste like sawdust and bark. Lignocellulosic biomass, rich in cellulose, hemicellulose, and lignin, is particularly advantageous due to its high carbon content and widespread availability. The chemical composition and moisture content of these feedstocks play a significant role in determining both the yield and quality of bio-oil; ideally, moisture levels should remain below 10%. In addition, feedstock selection is essential, as different biomass types exhibit varying pyrolytic behaviors, with higher cellulose content often leading to increased bio-oil production. Innovative options, including engineered microorganisms, expand our potential for bio-oil through pyrolysis.
Pyrolysis Process Overview
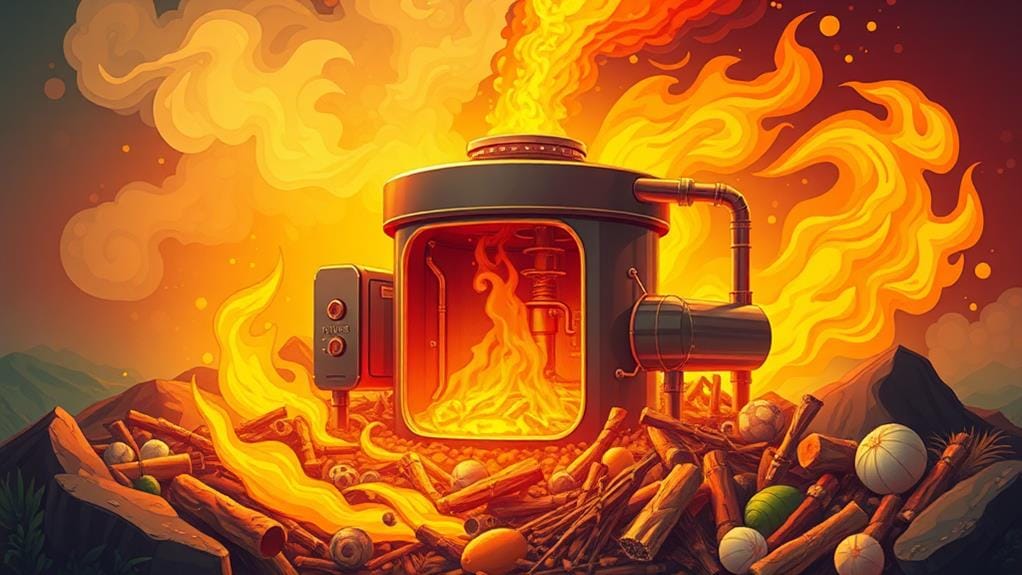
In this section, we'll provide a thorough overview of the pyrolysis process, focusing on the underlying mechanism that facilitates the thermal decomposition of lignocellulosic biomass and the various types of reactors utilized in the industry. By understanding the distinct categories of pyrolysis, particularly fast and slow pyrolysis, we can appreciate how different operational parameters, such as temperature and heating rate, influence bio-oil yields and the overall efficiency of the process. Additionally, we'll explore the implications of reactor design on the quality and quantity of the bio-oil produced, which is critical for optimizing production and economic viability.
Pyrolysis Mechanism Explained
One might wonder how pyrolysis effectively transforms biomass into valuable products. At its core, the pyrolysis process involves the thermal decomposition of lignocellulosic biomass in the absence of oxygen, typically at temperatures between 400-600 °C. This process can be categorized into fast pyrolysis, which utilizes high heating rates to maximize bio-oil yield, and slow pyrolysis, which produces more solid char.
- Elevated temperatures break down biomass into liquid products.
- Fast pyrolysis achieves up to 75 wt% bio-oil yield.
- The resulting bio-oil contains complex oxygenated organic compounds.
- Pyrolysis conditions play a significant role in determining quality and stability.
Types of Pyrolysis Reactors
When exploring the various types of pyrolysis reactors, we find that they greatly influence the efficiency and yield of bio-oil production. The three main types—fluidized bed reactors, fixed bed reactors, and entrained flow reactors—each present unique operational characteristics.
Reactor Type | Key Features |
---|---|
Fluidized Bed Reactor | Enhanced heat transfer, high bio-oil yields (up to 75 wt%) |
Fixed Bed Reactor | Simple design, lower bio-oil yields, scalability issues |
Entrained Flow Reactor | High temperature, short residence times, ideal for powdered biomass |
The choice of reactor considerably affects bio-oil yields and quality, with operating conditions differing based on the specific biomass feedstock used. Understanding these distinctions helps us improve the pyrolysis process for enhanced bio-oil production.
Types of Pyrolysis
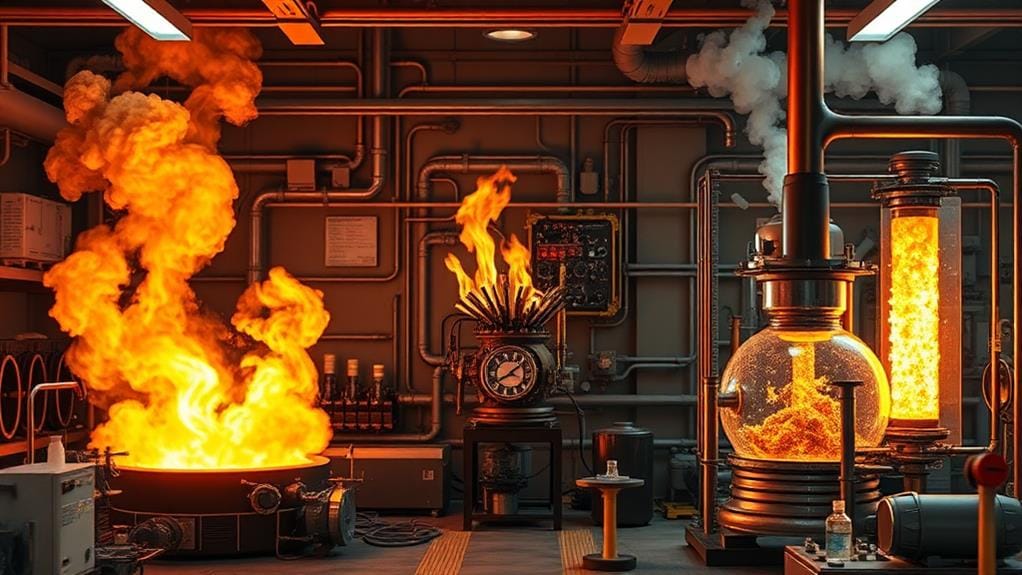
While both fast and slow pyrolysis serve the essential purpose of converting biomass into valuable products, they differ markedly in their operational parameters and outcomes. Fast pyrolysis operates at high heating rates, typically above 400°C, and short residence times of 2-10 seconds, yielding 60-75% bio-oil rich in oxygenated compounds. In contrast, slow pyrolysis employs lower heating rates and longer residence times to prioritize char production, affecting the bio-oil's composition.
- High yields of liquid fuels
- Varied reactor designs, like fluidized bed and fixed bed
- Distinct bio-oil characteristics based on conversion processes
- Temperature and particle size influence on quality
Understanding these types helps us optimize biomass utilization and enhance bio-oil production methodologies.
Bio-Oil Characteristics
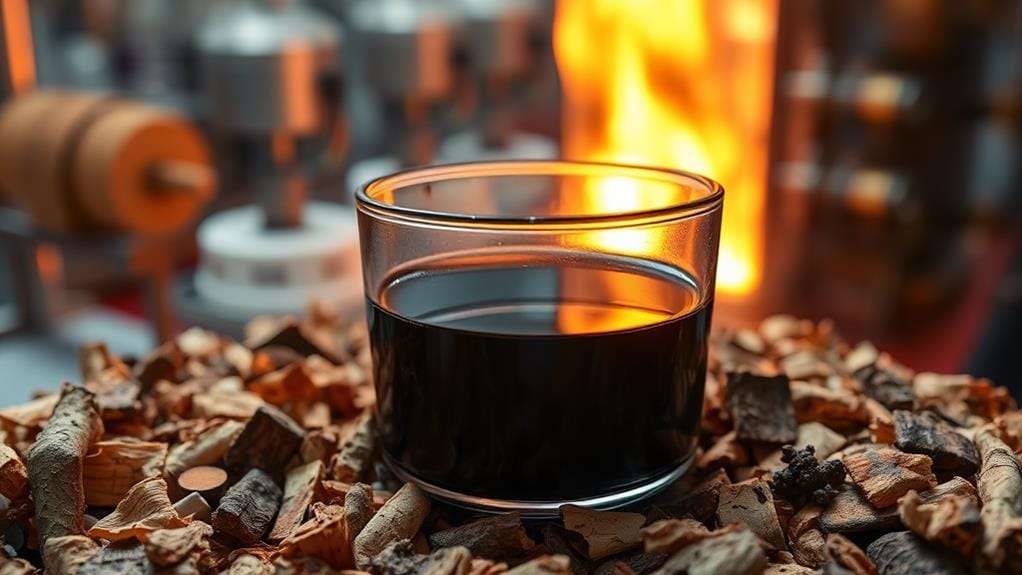
As we explore the characteristics of bio-oil, it is crucial to understand its complex chemical composition and how it compares to traditional fuels regarding energy density. Bio-oil, with its dark brown appearance and high oxygen content—often reaching up to 30%—presents unique challenges, including lower energy density and stability issues that require upgrading for effective utilization. Additionally, the wide range of carbon chain lengths, from C6 to C47, alongside the presence of various compounds such as phenols and ketones, complicates its application as a viable fuel source.
Chemical Composition Overview
Bio-oil, produced through pyrolysis, is a fascinating and complex mixture that presents unique chemical characteristics. It consists of various oxygenated organic compounds, leading to a high oxygen content of up to 30%, and contains 15-30 weight percent water, which impacts its viscosity and combustion properties.
- Low pH (around 2-3), primarily due to carboxylic acids, contributing to its corrosive nature
- Carbon chain lengths ranging from C6 to C47, influencing stability
- High viscosity, necessitating upgrading processes for improved fuel compatibility
- Diverse applications in energy production and as chemical feedstocks
These attributes underline the importance of bio-oil in advancing sustainable energy solutions, though they also pose challenges in refining and usability.
Energy Density Comparison
Although the energy density of bio-oil is lower than that of petroleum, it still presents a viable alternative for energy production, boasting a fuel value that ranges from 50-70% of conventional fuels. Its density of approximately 1 kg/L offers a cost-effective solution for transportation compared to solid biomass feedstocks. However, bio-oil contains 15-30 wt% water, impacting its viscosity and combustion capabilities, which necessitates upgrading to enhance its properties for engines and boilers. The carbon chain length, ranging from C6 to C47, indicates a diverse mixture of organic compounds that influence its applications. Additionally, reducing the high oxygen content, which can be as much as 30%, is essential for improving fuel stability and overall performance in combustion systems.
Applications of Bio-Oil
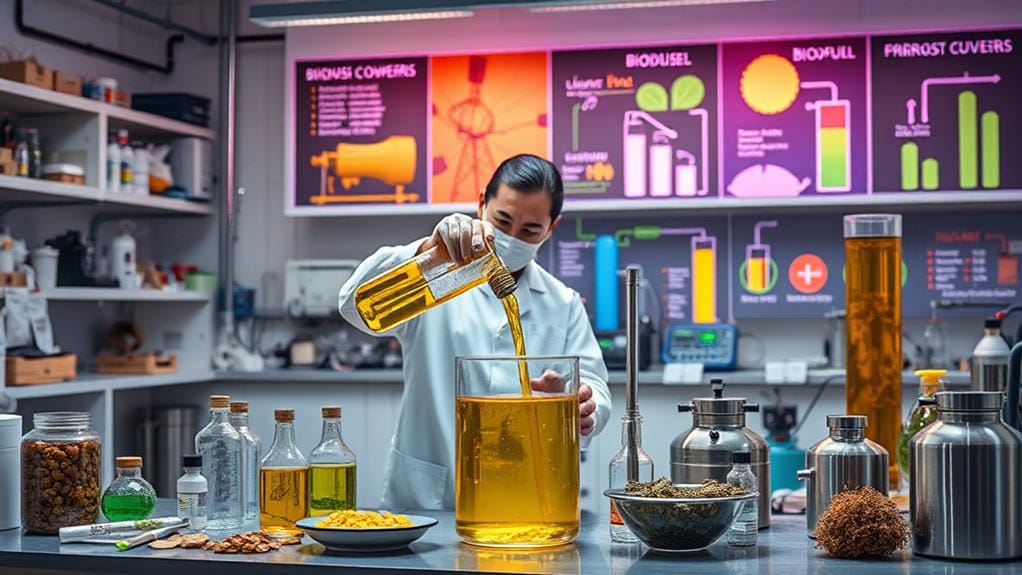
In exploring the diverse applications of bio-oil, we find it serves as a promising renewable alternative to fossil fuels. With its energy value being approximately 50-70% that of petroleum, bio-oil is well-positioned for various uses, including:
- Fuel for boilers and engines, enhancing energy generation
- Feedstock for chemical production, yielding essential chemicals
- Blending with diesel to improve engine performance
- Upgrading processes to enhance usability and reduce viscosity
These applications reflect bio-oil's versatility derived from biomass. Its potential as a viable fuel substitute, along with its capability to produce hydrogen and binders for electrodes, showcases the breadth of its applications in the renewable energy landscape. Together, we can harness bio-oil's benefits, paving the way for sustainable energy solutions.
Environmental Impact
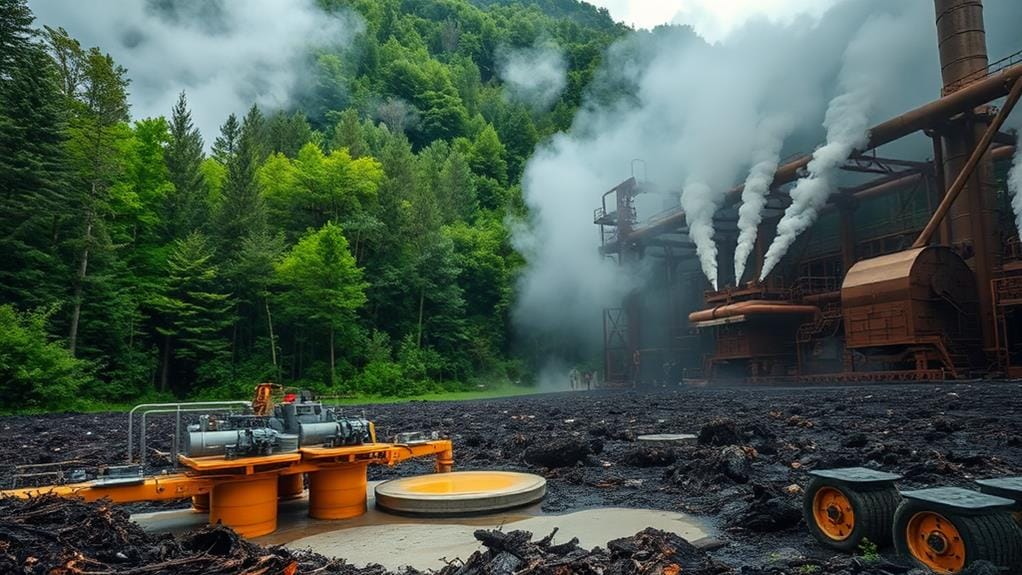
The production of bio-oil through pyrolysis not only offers a range of applications but also presents significant environmental advantages. By utilizing waste biomass, we can effectively divert organic materials from landfills, reducing carbon footprints associated with waste disposal. Furthermore, pyrolysis can lower greenhouse gas emissions by 60-90% compared to fossil fuels, making it a more sustainable option. Life cycle assessments indicate that when proper energy sources are used during biomass processing, greenhouse gas emissions can be minimized further. This process aligns with circular economy principles, as the valorization of exhausted biosorbents through thermochemical conversion supports sustainable waste management and regenerates materials for multiple reuse cycles, ultimately enhancing the environmental compatibility of bio-oil production and contributing to a greener future.
Future of Pyrolysis Technology
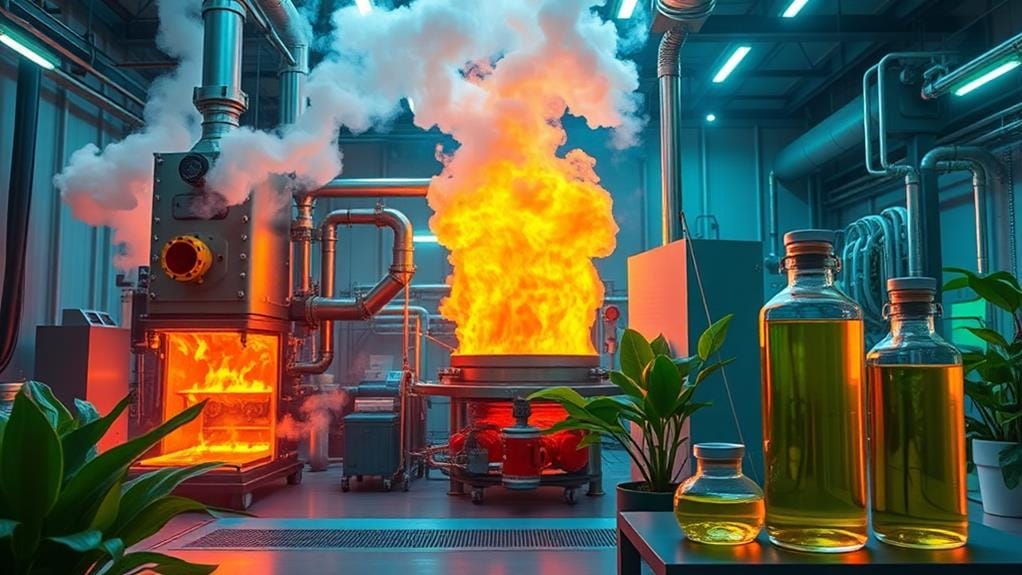
As we look toward the future of pyrolysis technology, exciting advancements promise to revolutionize bio-oil production. By improving process conditions, researchers can enhance bio-oil yield and quality, especially at ideal temperatures between 400-600 °C for various biomass types.
- Innovations in catalytic materials, like Ni2P on metal oxides, are improving hydrodeoxygenation efficiency.
- Integrating pyrolysis with other biomass conversion technologies maximizes energy recovery and supports circular economy principles.
- Advances in modeling fast pyrolysis processes deepen our understanding of reaction kinetics, essential for scaling up production.
- Research is also focused on minimizing GHG emissions and ensuring safe handling of heavy metals during biomass processing.
Together, we're paving the way for a sustainable future in bio-oil production.
Frequently Asked Questions
What Is Pyrolysis of Biomass for Bio-Oil?
When we explore biomass conversion, pyrolysis involves thermal decomposition, transforming renewable resources into valuable bio-oil. By selecting the right feedstock, we enhance product yield while promoting carbon neutrality and minimizing environmental impact for various industrial applications.
What Is Pyrolysis in Biofuels?
We're diving into the incredible world of the pyrolysis process! It's a fascinating biomass conversion technique that maximizes energy efficiency, transforming waste into renewable resources while minimizing environmental impact through careful feedstock selection and reactor design.
Is Pyrolysis Oil the Same as Bio-Oil?
We recognize that pyrolysis oil and bio-oil aren't exactly the same. The pyrolysis process yields bio-oil from biomass feedstocks, showcasing market potential and energy recovery while considering environmental impacts and advancements in production efficiency and chemical composition.
What Is the Importance of Biomass Pyrolysis?
Biomass pyrolysis is essential for sustainable energy; it transforms waste into renewable resources, reduces our carbon footprint, and minimizes greenhouse gases. Together, we can embrace thermal decomposition for better energy conversion and positive environmental impact.